Among the various solar fuels acting as energy vector of renewable sources, hydrogen from water photolysis is the most appealing output of artificial photosynthesis, given the abundance of water covering ∼71% of the Earth’s surface.
Solar energy conversion into hydrogen molecule via water photolysis is inspired by the first step of natural photosynthesis, which consists in breaking water under the energy input of sunlight. Similarly, artificial photosynthesis makes use of a photoabsorber semiconductor material driving the overall up-hill water splitting reaction into H2 and O2 molecules, through the following half reactions:
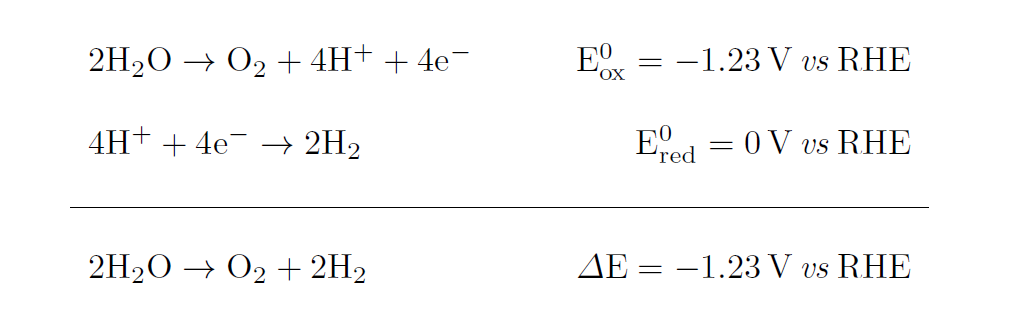
Water splitting is thus an endergonic reaction requiring a standard free energy ΔG0 = 237.2 kJ mol-1.
Photocatalytic water splitting through one-step photoexcitation requires the employed semiconductor to have a band gap energy large enough to split water, as well as suitable band edge positions straddling the reduction and oxidation potentials of water, i.e. the conduction band (CB) bottom of the semiconductor being located at a more negative potential than the H+/H2 energy level (0 V vs RHE), and the valence band (VB) top being more positive than the O2/H2O energy level (1.23 V vs RHE).
It is worth noting that, nevertheless, this thermodynamic requisite is in contrast with the need for the band gap to be as small as possible for the material to absorb a large portion of the solar spectrum. In fact, metal oxides which have been extensively studied as photocatalysts for water splitting generally possess VB located at ca. 3 V vs. RHE. This means that the band gap energy should be inevitably larger than 3 eV, with a consequent inactivity of the material in the most useful visible-light region of the solar spectrum. Therefore, it appears extremely difficult to find a photocatalytic system able to meet both the above-described requirements, making overall water splitting carried out on a single semiconductor material inefficient.
One possible solution to this issue consists in a two-step photocatalytic system known as ‘‘Z-scheme’’, in which two distinct photocatalysts with different band gap energies are combined in a so called ‘tandem cell’, in order to separately evolve H2 and O2. The photoanode carries out the water oxidation reaction, whereas the photocatode catalyses the hydrogen evolving reaction.
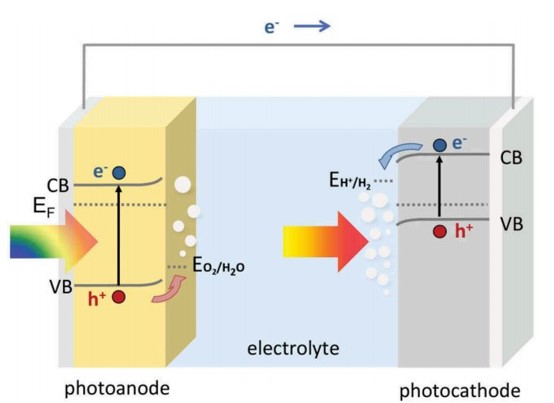
This system allows visible light to be utilized in a more efficient way with respect to the conventional one-step photoexcitation; since the two helf reactions of water splitting are carried out by two different photocatalysts it is possible to employ smaller band gap materials (the CB edge of the photoanode can be more positive than the H+/H2 potential and the VB edge of the photocatode can be more negative than the O2/H2O potential), which are active in a greater portion of the solar radiation and allowing, at the same time, photogenerated electrons/holes with stronger reduction/oxidation abilities to be accessible without the need of absorbing highly energetic photons.
Our research focus
Being water oxidation the much more challenging step of the overall water splitting process from both the thermodynamic and kinetics points of view, due to the involvement of four holes for one molecule of gaseous oxygen to evolve, in our group we focus our research on the development of efficient semiconductor-based materials acting as photoanodes.