Photocatalysis and photoelectrocatalysis play a crucial role in advancing the research towards more sustainable and renewable forms of energy, by efficiently exploiting the light irradiated by the Sun and transforming it in chemical energy, i.e. by storing it in chemical bonds.
Solar Energy Conversion
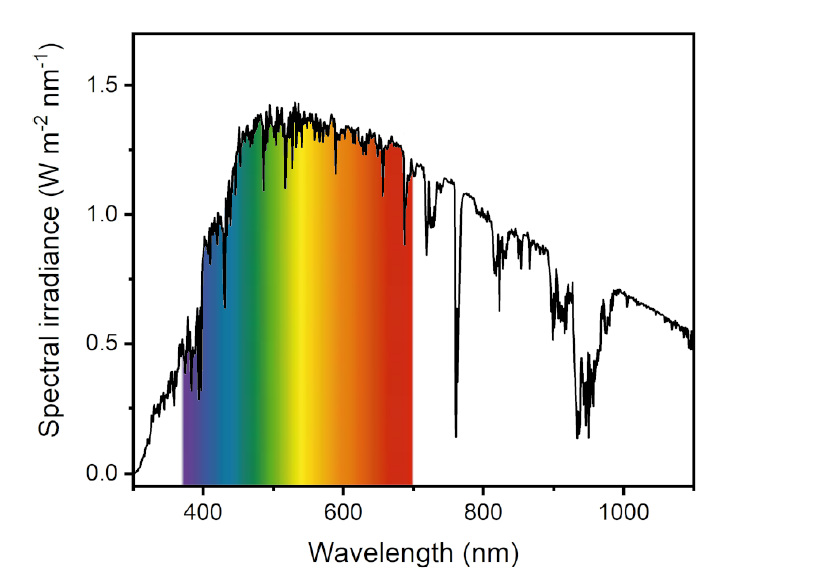
Finding new, environmentally sustainable sources of energy is a compelling challenge. Sunlight is a clean and renewable energy source and provides our planet with an intense flux of high energetic photons, that are capable of promoting up-hill reactions, such as the photosyntetic fixation of CO2. Moreover, since more energy from sunlight strikes Earth in an hour that all the energy consumed by humans in an entire year, much research is focused on how to capture, convert and store this type of energy.
In this context, solar energy storage in the form of chemical bonds of high energy molecules, such as hydrogen (H2), via artificial photosynthesis has proved to be an attractive route to undertake for pursuing the energy transition from fossil fuels to renewables.
Photocatalysts are materials which are capable of exploiting solar energy by absorbing solar incident light and promoting a chemical reaction, thus resulting in solar energy conversion, where the electromagnetic energy is converted into chemical energy. When the photocatalyst is a solid this process is defined heterogeneous catalysis.
Heterogeneous Photocatalysis
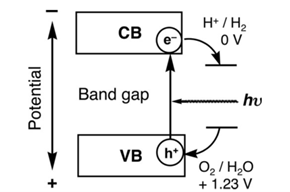
The most common photocatalysis are semiconductor materials. Semiconductors possess a void energy region between the top of the valence band and the bottom of the conduction band, called band gap, Eg. The activation of a semiconductor photocatalyst is achieved through the absorption of a photon of ultra-band gap energy, which results in the promotion of an electron from the valence band into the conduction band, e–CB, and in the concomitant generation of a hole in the valence band, h+VB. The reaction of either the photopromoted electron with a reducible adsorbed substrate and/or the hole with an oxidizable adsorbed species can subsequently occur.
The probability and the rate of such charge transfer processes depend on the position of the conduction and valence band edges and on the redox potentials of the adsorbed species. The figure here below shows the band gap values of different semiconductors and their position on the electrochemical scale. A substrate can successfully interact only with some semiconductors: it is necessary that the electrochemical potential value of the electron acceptor is more positive than the semiconductor conduction band potential, and that the electron donor potential is more negative than that of the semiconductor valence band. A photocatalytic reaction can take place only under such conditions.
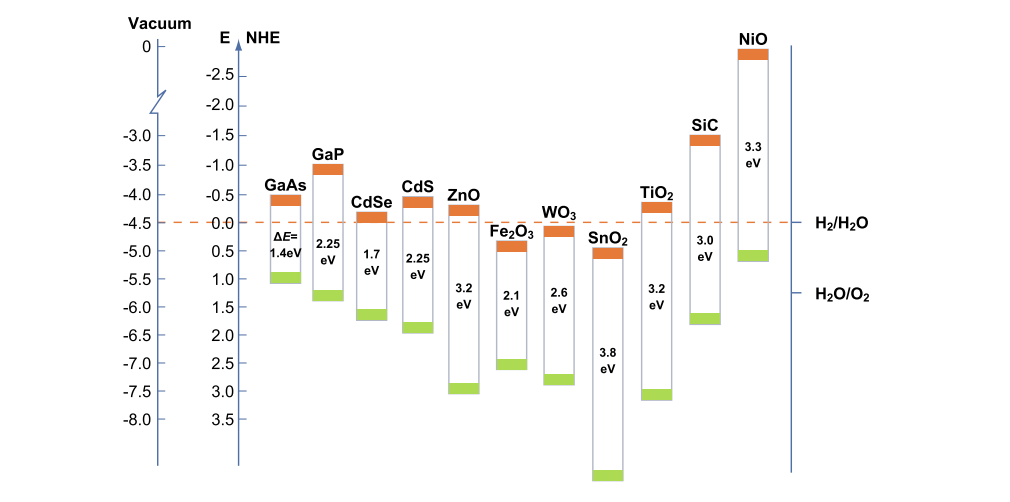
The reported band diagram also suggests that non-oxide semiconductors exhibit highly negative ECB, which makes them particularly suited to reduce water, whereas oxide semiconductors, with typically highly positive EVB, are mainly employed to carry out water oxidation.
Generally, the characteristic of a suitable semiconductor to act as an efficient photocatalyst include:
- VB and CB edges energetic enough to drive the desired reaction
- High chemical stability
- Relatively small band gap, allowing the material to maximise the absorption of visible light
- Fast surface kinetic for the desired reaction
- A slow recombination of photogenerated electrons and holes
In our research group we focus on the optimisation of metal oxide semiconductors in order to achieve better performing photocatalysts to be employed for water remediation and/or water splitting applications.
Here below you can find our research lines and learn more about the systems we study and the optimisation strategies employed to maximize their performances.
Photoelectrocatalysis (PEC)
We prepare thin film electrodes and evaluate their performances in PEC cells under simulated solar irradiation.
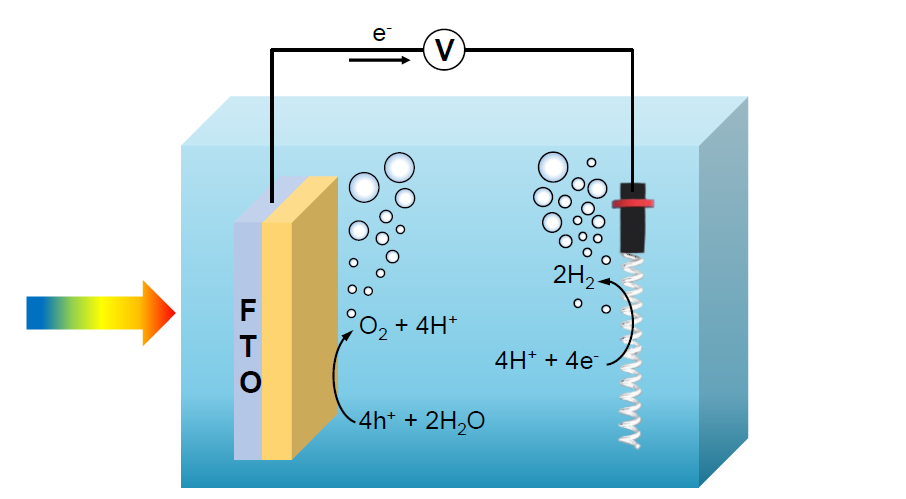
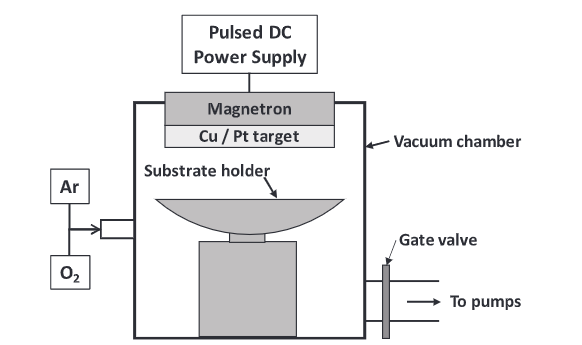
Industrial Applications